Applications of Organ-on-Chip Technology in Biomedical Research and Drug Development
Organ-on-chip (OoC) technology finds extensive applications in academia, pharma, biotech as well as cosmetic industry, spanning toxicology, immunology, gene therapy, and cancer research and many more. In academia, it facilitates the development of models closely resembling human physiology, aiding in understanding disease and infection mechanisms. In drug development, it enhances the assessment of drug efficacy and toxicity pre-clinically, thus refining drug quality before clinical trials. Offering superior predictive accuracy, it streamlines drug development, reduces costs, and accelerates time-to-market. Moreover, it enables the creation of novel disease models, explores new biological pathways, and holds promise for personalized medicine by modeling patient-specific responses to treatment using biopsied cells. This blog aims to list some of the application and research topics in which OoC models have shined.
Organ-on-Chip in Drug Development

Drug development is probably the field that could experience the most revolutionary shift by systematically implementing organ models into their preclinical pipelines. In a 10 to 15 year process, typically only 1 out of 5,000 – 10,000 potential drugs from drug discovery will reach approval by the health authorities (Rousseaux 2023 et al.). According to Sun 2022 et al. the 90% of drugs failing after clinical trial phase 1 are due to lack of clinical efficacy (40%–50%), unmanageable toxicity (30%), poor drug-like properties (10%–15%), and lack of commercial needs and poor strategic planning (10%). Conversely, this means that about 40 – 90% of cases could be avoided by using human relevant in vitro models in preclinical trials instead of animal models or too simplistic 2D in vitro models (learn more here). OoC models offer a revolutionary alternative, providing researchers with highly predictive platforms to assess drug responses with greater precision. Read more on the specific application for drug development below.
Lead optimization
Especially in the later stages of preclinical development during lead optimization when only a few selected lead targets are left is where OoC models will shine. Apart from a few exceptions, OoC technologies are typically not good at screening a lot of compounds in a high-throughput manner, but they are exceptional great at delivering in depth data on a select few. To learn which kind of data such models can provide, take a look at the subchapter below. Important to note is that the choice of biochip material, on which organ tissues are cultured in, is of high importance for applications in drug development. Since PDMS tends to adsorb a lot of compounds and interferes with cell growth, biochips made of such material should be avoided (learn more here)
Drug safety profiling
Toxicology helps to determine to which degree a compound negatively impacts the human body and its organs. Although animal models offer the advantage of predicting the metabolization of a drug throughout a whole organism, they lack human relevance and cannot faithfully predict how a human body would react within clinical trials. Particularly, liver- and kidney-on-chip models are interesting for toxicity profiling as they can indicate hepatoxicity or nephrotoxicity at an early stage, respectively. Although OoCs are not ideal in reproducing the metabolic pathways of a whole organism, multi-organ models are able to bridge this gap by combining multiple organs on one biochip. They thereby provide a tool, combining drug administration routes either through lung or the intestine, with typical organs metabolizing the drugs such as kidney or liver (image 2).
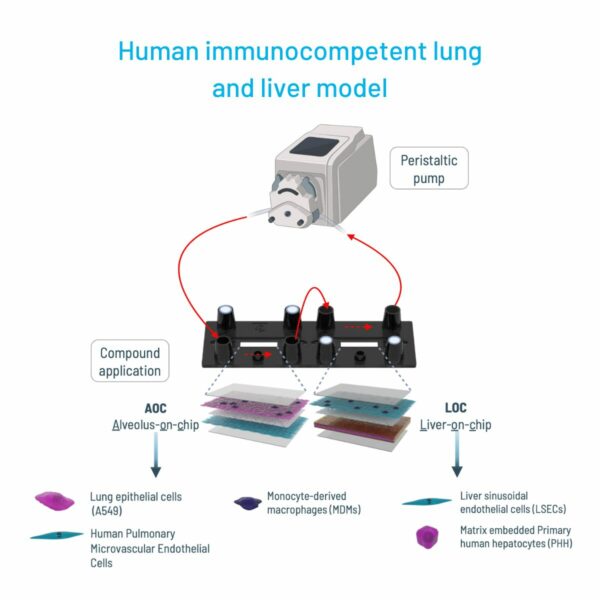
Pharmacodynamics & pharmacokinetic studies
OoC platforms facilitate detailed investigations into drug absorption, distribution, metabolism, and excretion (ADME) and the biochemical effects a compound could have on an active site in the human body relative to the concentration of the drug. By accurately recapitulating organ-specific functions and interactions, these models provide data for pharmacokinetic profiling, guiding dose optimization and formulation strategies.
Antibody safety and target profiling
As much as the immune system is involved in regulating the host’s defense against pathogens and cancer cells, it can also cause harmful tissue damage when the immune response is exaggerated. (Wang 2023 et al.). Assessing such harmful effects early on during preclinical trials can prevent bringing potentially harmful immunomodulatory antibodies or other therapies into clinical trials. A potent tool for such investigations are immunocompetent organ-on-chip models that allow to test for such harmful effects in an in vitro system. An example is a 3D microphysiological model of the human blood vessel that we developed at Dynamic42. This model was tested using TGN1412, an antibody deemed save and highly effective in pre-clinical studies conducted on macaques. Clinical trials, however, had to be terminated after six volunteers experienced a life-threatening CD4 T cell/IL2-driven cytokine storm and blood vessel inflammation followed by vascular leakage. When tested in our in vitro model, TGN1412 induced the release of IL-2 from CD4+ T cells, leading to a breakdown of the endothelial barrier and subsequent vascular leakage.
Cholestasis
In cholestasis, bile flow is obstructed between the liver cells, where bile is produced, and the duodenum, the initial part of the small intestine. This interruption leads to the escape and buildup of bilirubin, a waste product from the breakdown of old or damaged red blood cells, in the bloodstream (msd manuals). Typically, bilirubin combines with bile in the liver, travels through the bile ducts to the digestive system, and is expelled from the body. While most bilirubin exits through stool, a small portion is excreted in urine. Especially, 2-organ models combining the liver and intestine will allow studying cholestasis in great detail.
Tissue Barrier Models – Transport Studies
Understanding barrier function and monitoring barrier integrity is fundamental for unraveling the complexities of physiological processes and disease mechanisms in the human body. Barriers play a particularly crucial role in drug discovery as they are often responsible for drug transport into the cell or are part of the drug target mechanism. However, traditional models, such as 2D cell cultures or animal models, often have fallen short in relevance, reproducibility, and translatability. This has spiked the emergence of microphysiological system, also called organ-on-chip models, replicating human organs in vitro. Those platforms provide a much more physiologically relevant environment for studying barrier functions.
Disease Modeling
OoC technology enables the creation of sophisticated disease models, including multiple cell and tissue types, vascularization, and immune cells. They thereby can aid in shedding light on disease mechanisms and facilitating the development of targeted therapeutics by providing human relevant data. A good example are autoimmune diseases, which can affect almost all organs (Mello 2019 et al.). Triggers are complex, but usually involve a combination of genetic and environmental factors. Due to their complex nature, most, inflammatory diseases cannot faithfully be replicated in animal models, creating the need for physiological human in vitro models to advance research in this field. Immunocompetent organs-on-chip offer a potent model to study chronic inflammatory processes, paving the way for a deeper understanding of these diseases and the development of targeted therapies.
Organ-on-Chip in Biomedical Research
Beyond drug development, organ-on-chip technology plays a pivotal role in advancing biomedical research, offering innovative platforms to study disease pathogenesis, host-pathogen interactions, and tissue physiology.
Disease Modeling
Similarly to drug development, OoC can also serve as an advanced human in vitro model to study diseases in an academic setting. OoC models can faithfully replicate the pathophysiology of various diseases, providing researchers with powerful tools to unravel complex disease mechanisms and test potential routes for interventions.
Infection Modeling
Particularly challenging is the research going into infectious diseases, due to a lack of models that can faithfully reproduce the complexity and physiology of a human host’s response to a pathogen. OoC technology aims to fill this gap by providing a model system that allows for formation of human tissue organized like in vivo but also by including immune components, a microbiome and pathogens of various kinds. They thereby offer a dynamic platform to study infectious diseases and to develop targeted interventions by enabling a flexible set-up of the model (Image 3).
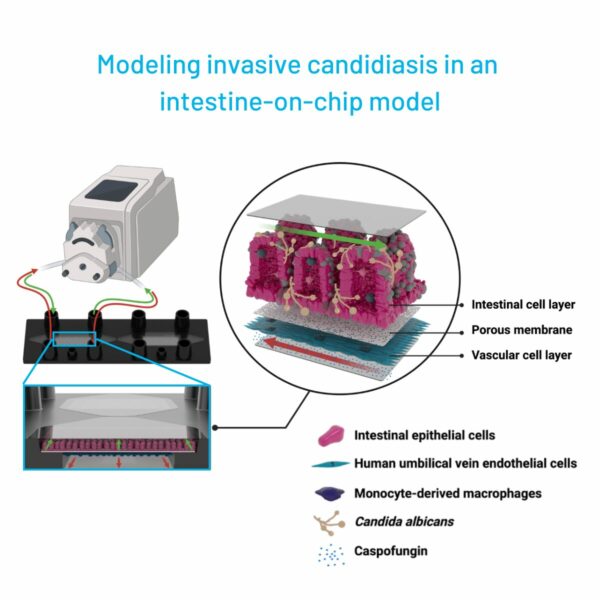
Host-microbiome interaction
The human intestine harbors a diverse microbiome crucial for metabolic and digestive functions. OoC models are capable of including such organisms provide a platform to study the protective effect of the microbiome as well commensal organisms and the conditions under which they turn pathogenic. A great example is an intestine-on-chip pre-colonization with probiotics which mitigated damage induced by the fungus Candida albicans (Maurer et al. 2019). Furthermore, they allow researchers to study the effect of treatments in a more physiological environment (Kaden et al. 2024).
Immune cell interaction
The immune system is a critical component of the human body, playing a vital role in protecting against infections, eliminating diseased cells, and maintaining tissue homeostasis. Replicating the immune response in conventional 2D-in-vitro models often is a limiting factor. OoC models on the other hand are able to include circulating and resident immune cells and thereby recapitulating the human immune response in vitro. This is crucial for application in modelling of inflammatory as well as chronic diseases, to study tissue-resident immune cells and their impact on organ function, accurate disease modelling, cancer research and many more (learn more here).
Conclusion
In conclusion, OoC technology represents a paradigm shift in biomedical research and drug development, offering the exciting capabilities to replicate human physiology, model disease processes, and assess drug responses with high accuracy. With its potential to sustainably change drug discovery, improve preclinical testing, and advance personalized medicine, OoC technology stands at the forefront of innovation, poised to shape the future of healthcare. As researchers continue to refine and expand upon these platforms, the possibilities for discovery and innovation are limitless, promising new insights into human biology and novel solutions to combat disease.
More interesting articles:
Blog
The PDAC-on-Chip – A New Dimension in Pancreatic Cancer Research
A new biochip replicates key aspects of the PDAC microenvironment, providing a powerful tool for drug development and reduction of animal testing.
Read MoreBlog
What is TEER? – Trans-Epithelial Electrical Resistance Assay
Here we explore TEER assays as an excellent method to determine barrier, and thereby epithelial and endothelial tissue integrity.
Read MoreBlog
Exploring Barrier Function with TEER Assays in Organ-on-Chip Models
In this blog we will delve into the significance of organ models in studying barrier function, focusing on TEER-assay, exploring its essential features, and showcasing examples of groundbreaking research in various organ systems.
Read More